The History of Gamma-ray Astronomy
Long before experiments could detect
gamma-rays
emitted by cosmic sources, scientists had known that the Universe should be
producing such high energy photons. Hard work by several brilliant scientists had shown us
that a number of different processes which were occurring in the Universe
would result in gamma-ray emission. These processes included cosmic ray
interactions with interstellar gas,
supernova
explosions, and interactions of energetic electrons with
magnetic
fields. In the 1960s, we finally developed the ability to actually detect
these emissions and we have been looking at them ever since!

|
Gamma-rays coming from space are mostly absorbed by the Earth's
atmosphere. So gamma-ray
astronomy could not develop until it was
possible to get our detectors above all or most of the atmosphere,
using balloons or spacecraft. The first gamma-ray telescope carried
into orbit,
on the Explorer XI satellite in 1961, picked up fewer than
100 cosmic gamma-ray photons. These appeared to come from all
directions in the Universe, implying some sort of uniform
"gamma-ray background". Such a background would be expected
from the interaction of cosmic rays (very energetic charged particles
in space) with gas found between the
stars.
|

Additional gamma-ray experiments flew on the OGO, OSO, Vela, and Russian
Cosmos series of
satellites.
However, the first satellite designed as a
"dedicated" gamma-ray mission was the second Small Astronomy
Satellite (SAS-2) in 1972. It lasted only seven months due to an
electrical problem, but provided an exciting view into the high-energy
Universe (sometimes called the 'violent' Universe, because the kinds of
events in space that produce gamma-rays tend to be explosions, high-speed
collisions, and such!). In 1975, the European Space Agency
launched a similar satellite, COS-B, which operated until 1982. These
two satellites, SAS-2 and COS-B, confirmed the earlier findings of the
gamma-ray background, and also detected a number of point sources.
However, the poor
resolution of the instruments made it impossible to identify most of these
point sources with individual stars or stellar systems.

|
Show me a movie about Dr. Compton
|
The man seen in this image is Dr. Arthur Holly Compton. He won the Nobel
Prize for Physics in 1927 for his work on the scattering of high-energy
photons by electrons - which is something very basic to most
gamma-ray
detection techniques. The Compton Gamma-Ray Observatory was named in his
honor after it went into
orbit in April 1991.
It continued to operate until June 2000, giving scientists the best look they have ever
gotten of the gamma-ray universe.
So what are gamma-rays and what can they tell us about the cosmos?
Gamma-rays are the most energetic form of
electromagnetic
radiation, with over 10,000 times more energy than
visible light
photons. If you
could see gamma-rays, the night sky would look strange and unfamiliar. The
familiar sights of constantly shining
stars and galaxies
would be replaced by something ever-changing. Your gamma-ray vision would peer
into the hearts of
solar flares,
supernovae,
neutron stars,
black holes, and
active galaxies.
Gamma-ray astronomy
presents unique opportunities to explore these exotic objects. By exploring
the universe at these high energies, scientists can search for new physics,
testing theories and performing experiments which are not possible in
earth-bound laboratories.
 |
Show me a movie about gamma-ray astronomy!
|
Gamma-Ray Observing Platforms
Most gamma-rays are absorbed by the Earth's atmosphere. Thus, cosmic
gamma-rays are typically observed from high-altitude balloons and
satellites.
Balloons
Balloons are often the ideal vehicle for science payloads. At energies
greater than about 30 keV, hard (more energetic)
X-ray and gamma-ray
astronomy can be done
from balloons. At these high photon energies, being above 99.7 % of the Earth's
atmosphere (which is where you are with a balloon float altitude of 40 km) is
as good as being above 100 % of the atmosphere!
Balloon-borne hard X-ray and gamma-ray imaging telescopes provided the
first images of the
sky in the energy range 20-1000 keV. They discovered
black hole
candidate sources in the galactic center region, first imaged the cobalt-decay
gamma-rays from the spectacular supernova SN 1987A, and provided the first
capability to localize (and thus study) high-energy sources for comparison
with more detailed lower-energy X-ray observations.
In the past, balloon flights typically remained at float altitude from
several hours to one or two days, at best. Nowadays, however, there are
balloons called Long-Duration Balloons (or LDBs) which typically stay aloft
for 3 weeks or more. They can carry a payload of over 2000 kg, with active
experiment areas of up to 30 m2! Perhaps just as importantly in
the modern era, balloon experiments are relatively inexpensive (when compared
to satellite missions). Ballooning today is not the same as ballooning in the
past!
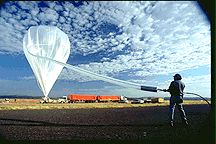
Filling the balloon for a flight of the
GRIP payload
Satellites

Satellites provide an excellent platform from which to observe cosmic
gamma-rays. One reason is that the intensity, or flux, of cosmic gamma-rays
arriving at the Earth is very low when compared to other sources of
high-energy photons. Thus, staying in
orbit observing for
many months or years allows scientists to gather more and more cosmic
gamma-rays. The more "real" gamma-rays they collect, the better
their data analysis can be. Since 1961 when the first satellite went into
orbit carrying a detector sensitive to energies greater than 50 MeV, many
satellites have flown to observe cosmic gamma-rays. While the first satellite,
Explorer-11 detected only 22 cosmic gamma-rays, 621 events attributable to
cosmic gamma-rays were detected by the OSO-3 satellite in 1967. Since then,
the sensitivity
of the detectors has increased by factors of 1000! This has allowed
scientists to make many fascinating discoveries in the high-energy universe.
For example, they have mapped the diffuse gamma-ray background, found
gamma-ray pulsars,
and discovered the still-unexplained cosmic gamma-ray bursts!
NASA's Wind satellite, pictured on the right, has 2 instruments which have
been monitoring these bursts since 1994.
Targets of Gamma-ray Observations
Observing the Universe in gamma-rays allows us to examine things which are
happening that cannot be seen with ordinary telescopes and, yet, which are
very important in helping us to understand how
matter and
radiation
interact with each other. This is especially true for understanding their
interaction under extreme conditions, such as where temperatures are hundreds
of millions of degrees, matter is very dense, or
magnetic
fields are very strong.
Some specific targets include:
|